What is Quantum Photonics?
My Master’s studies at the University of Toronto focused on the emerging field of quantum photonics, which exploits exotic quantum-mechanical attributes of light (such as entanglement, superposition, and ‘quadrature squeezing’) to outperform existing conventional technologies. This makes it possible to create:
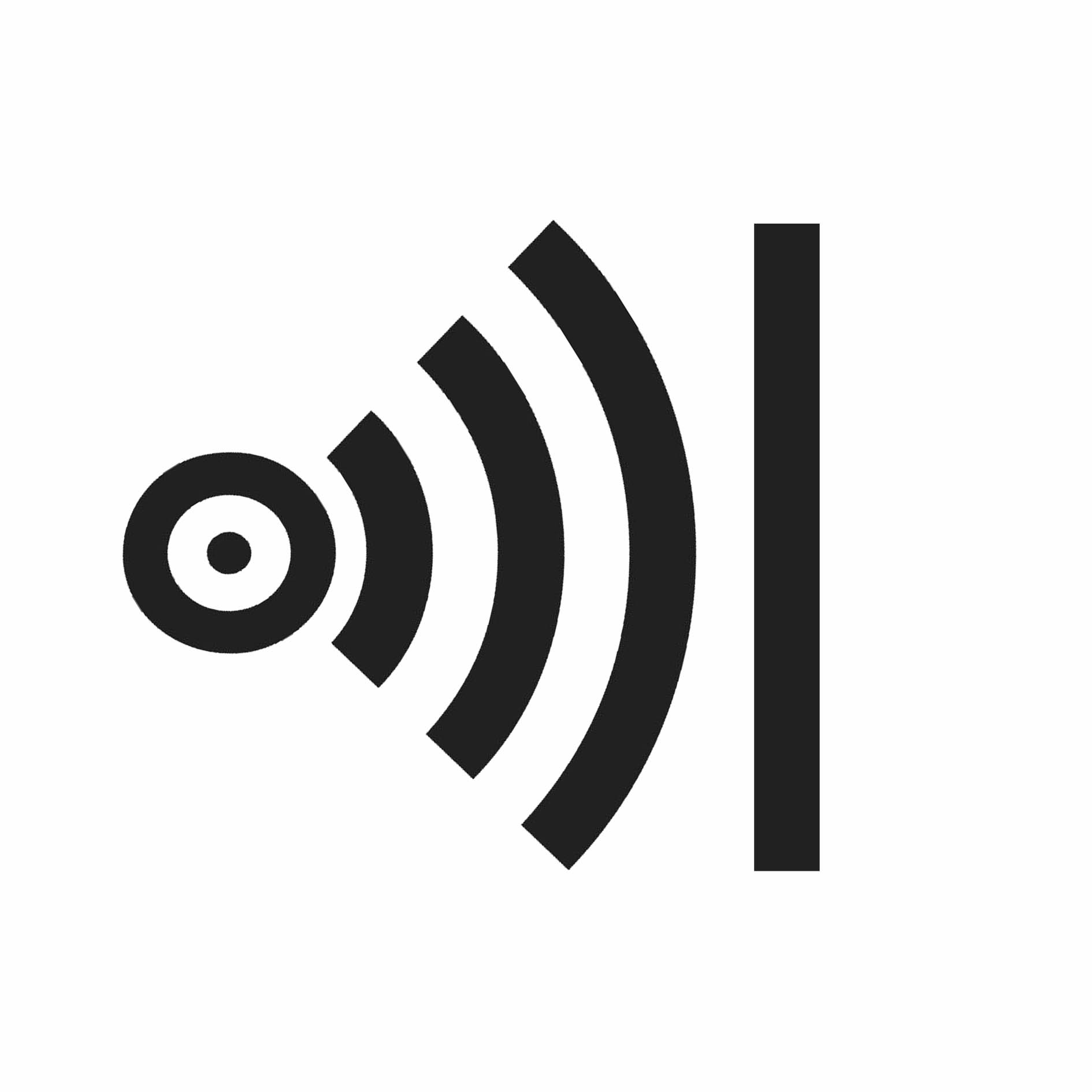
quantum-enhanced sensors that beat classical noise limits to provide superior sensitivity
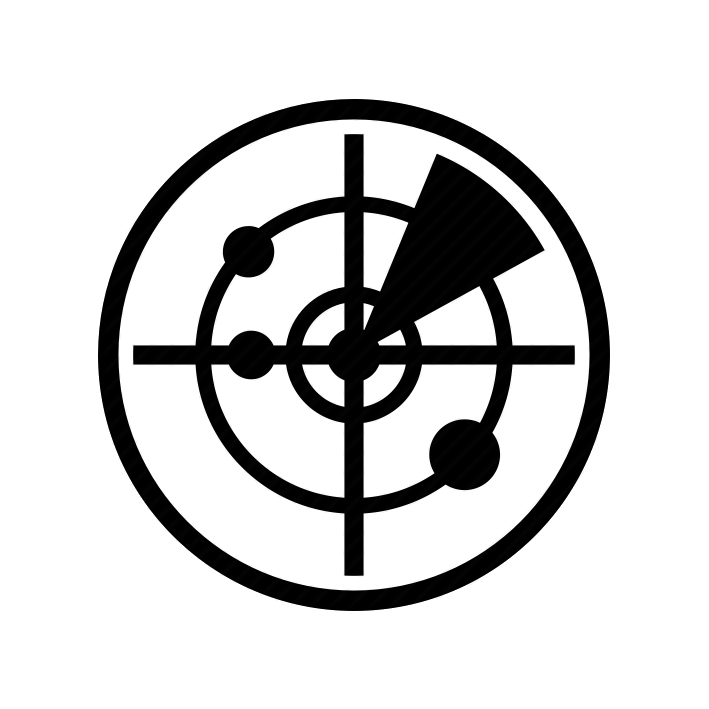
quantum LIDAR that has immunity to signal jamming and is better at detecting faint signals in noisy environments
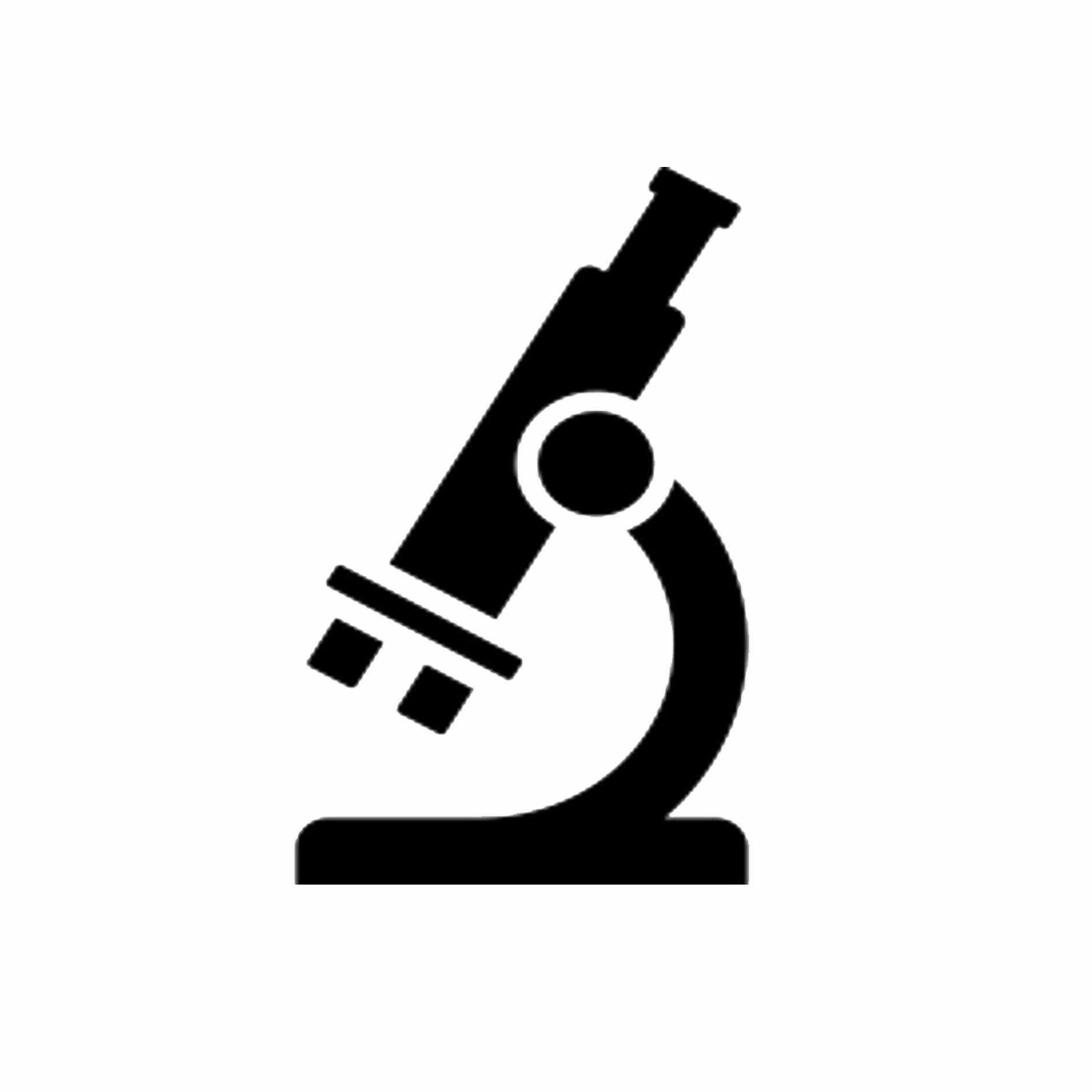
optically efficient high-performance microscopes that expose photo-delicate specimens to less light during imaging
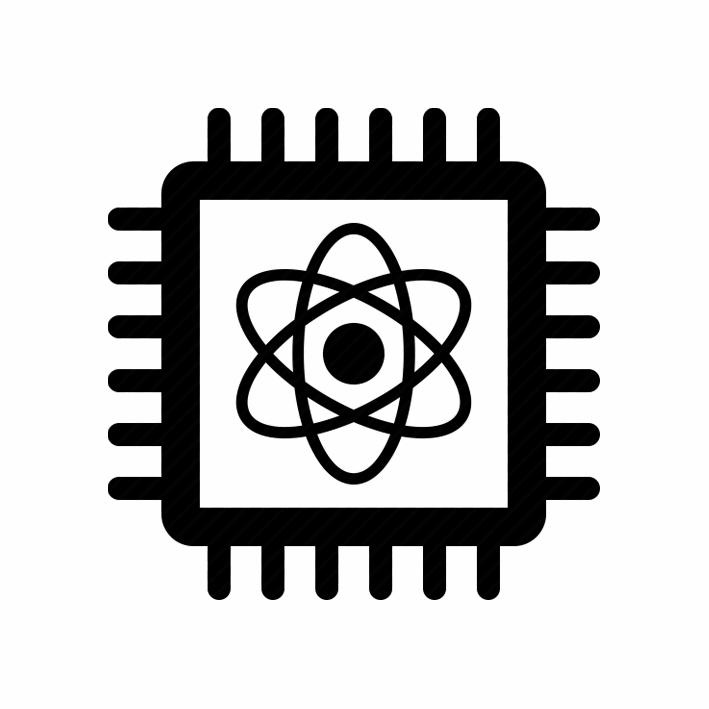
building-blocks for quantum computing infrastructure, such as quantum data routers and photonic quantum processing units
Why Integrated?
A major challenge facing quantum photonics today is that it typically lacks the portability, scalability, ruggedness, and easy operation required to make it a useful technology. One of the goals of our research team was to solve this problem by developing practical chip-based quantum photonic technologies that leverage the integrated-optics approaches used so successfully by the telecommunications industry. This would help bring quantum photonics out of the lab and into real-world applications.
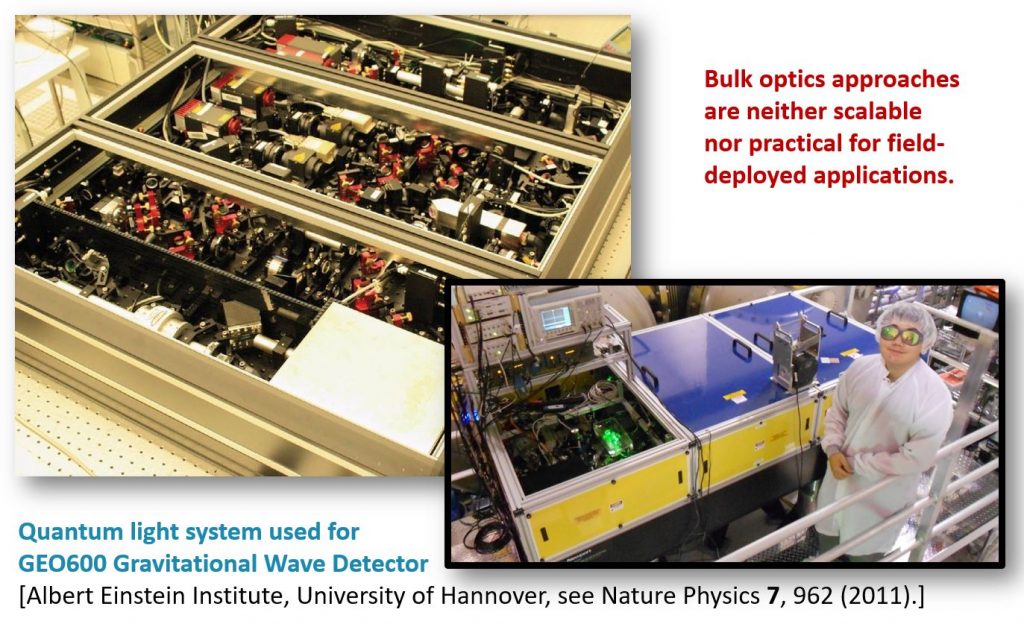
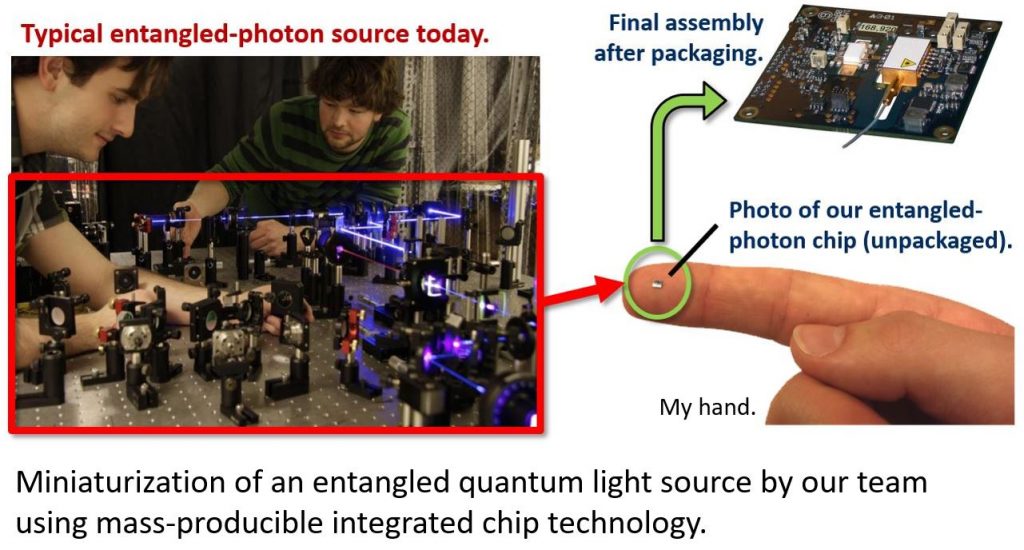
My Thesis Objectives
Entangled photon pairs are a key ingredient of many quantum optics technologies. Building upon our team’s expertise for generating this exotic type of light on a photonic chip, my work concentrated on how to best manipulate and utilize it. Two of the questions I focused on during my thesis were:
- How can we deterministically separate arbitrary photon pairs into different optical waveguides without compromising their quantum entanglement? These ‘twin’ photons are often generated within the same waveguide, but must be routed to separate parts of the chip for independent manipulation.
- Can the integrated setting provide any unique capabilities for manipulating and processing entangled-photon states that are not possible using conventional bulk optics?
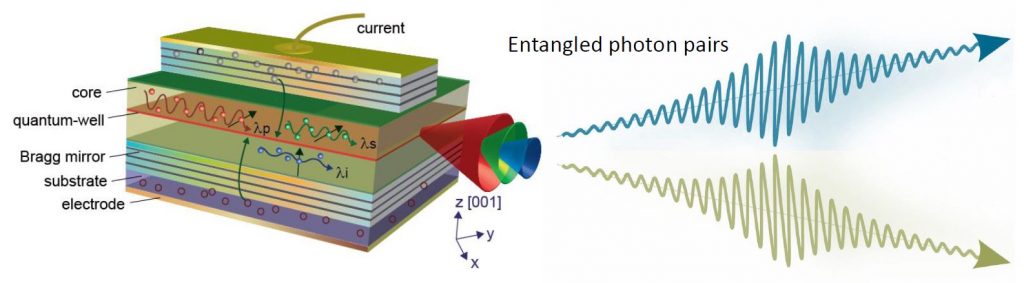
Schematic of our team’s all-integrated entangled-photon source.
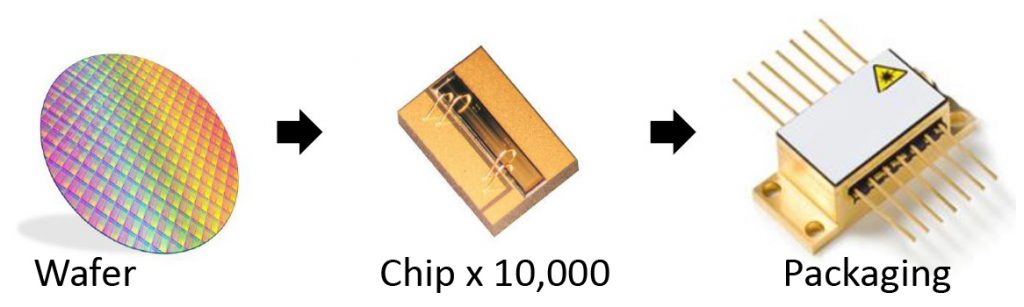
The entangled-photon chips are mass manufactured as a semiconductor wafer, subdivided into tiny dyes, and can be electrically packaged for easy operation.
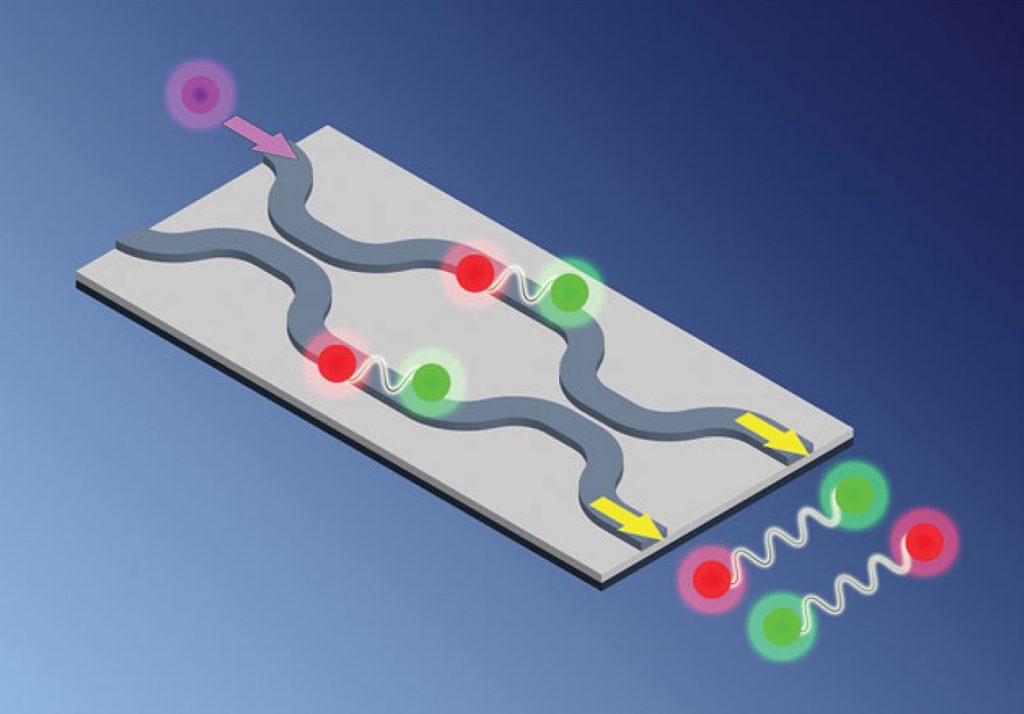
Artistic rendering of deterministic entangled-pair separation, wherein two indistinguishable sources of photon pairs are coherently pumped and quantum-mechanically interfere at an on-chip mode coupler.
Separating Twin-Photons
To deterministically separate entangled photons with arbitrary properties, I made use of a process called quantum interference, wherein the quantum amplitudes associated with the photons leaving together from the same output waveguide are engineered to cancel out.
I found that a phenomenon known as dispersion (which is much stronger on a chip than in bulk optics) could have a significant impact on the way two-photon states behave and interfere, which can frustrate our efforts to separate them. However, I showed that these issues could largely be mitigated with appropriate engineering. My findings were published in Laser & Photonics Reviews and can be read here.
Designing Photonic Chips
My Master’s thesis also involved the design of integrated photonic circuits for experimentally demonstrating my findings. The design process included the creation of device physics models using MATLAB and Python scripts, and finite-element analysis simulations of the on-chip light propagation. Selection of appropriate material parameters (such as layer thicknesses and materials) drew upon my knowledge of micro-fabrication processes. A prototype batch of path-entangled photon pair sources that I designed was fabricated by our team for testing, and is depicted in the images below. Further details can be found in my full thesis here.
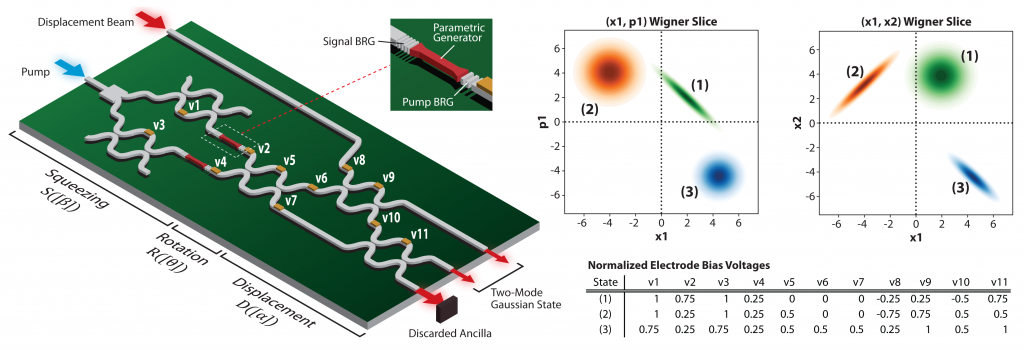
A programmable photonic quantum circuit for generating arbitrary two-mode Gaussian states. See the ArXiv preprint here.
What's Next for Quantum Photonics?
In the long run, quantum photonics is expected to complement the solid-state quantum computing infrastructure, either by facilitating the creation of hybrid computing platforms, or by allowing remote quantum computing clusters to link their resources together by entangling them. Meanwhile, several countries, including China and Canada, are pursuing space deployment of entangled photon sources to establish quantum-secure encryption networks. In the shorter term, quantum photonics can have more immediate benefits for high-performance sensing and imaging, where it can provide enhancements over classical technologies.
In late 2016, I co-founded a company called QuWare together with Professor Amr Helmy at the University of Toronto to begin commercializing some of the technologies developed by our lab. The next revolution in photonics technology is just around the corner…
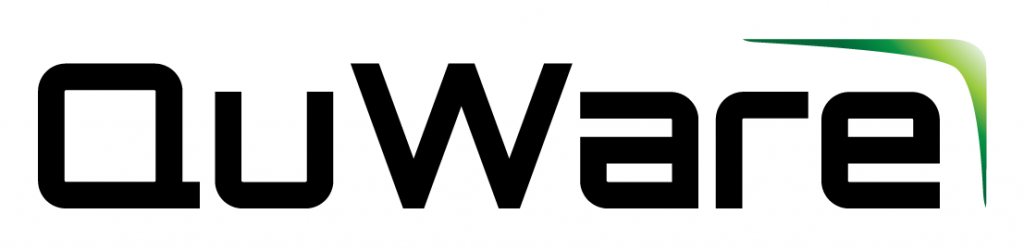
Check out some of my other past projects!
I’ve worked on technical projects in a variety of fields. Here are some highlights: